Distributed massive MIMO is a breakthrough in delivering a high-quality 5G experience indoors
05 March 2021 | Research
Article | PDF (3 pages) | Next-Generation Wireless Networks
The use of mobile data services has increasingly been shifting towards indoor environments in recent years because users have come to rely on the cellular network for communications, business and entertainment wherever they are. This trend will be intensified in the 2020s because 5G has many additional capabilities over 4G, such as support for massive device densities and multi-gigabit speeds. These capabilities will drive new usage patterns, many of which will be concentrated indoors. However, achieving the very high capacity, reliability and performance that these new services require is particularly challenging inside buildings, and traditional solutions such as cell splitting are reaching their limits.
In this article, we will assess an emerging approach to this challenge called distributed massive MIMO. This approach will extend massive MIMO technology from outdoor to indoor environments for the first time (with antenna configurations up to 64T64R), significantly improve the capacity and quality of experience for users indoors and therefore enhance the 5G business case for operators.
A high-quality indoor experience will be critical to the 5G business case
Mobile networks were originally conceived as primarily outdoor systems, to be used when people were away from their homes or office phone lines. They have steadily become the primary network for many users, regardless of where they are located, and this has created the challenge of adapting the networks to deliver the same quality of experience deep inside a building as they do outside. The demands of an indoor environment are very different to those of an outdoor one: rapid mobile hand-off is less important, but stationary users are likely to consume more video or other high-bandwidth services, for example. There are fewer issues with changes in weather or obstacles, but the density of devices will often be far greater.
These differences mean that the indoor cellular network must have very different characteristics and priorities from its outdoor counterpart, but a seamless experience must be maintained when moving between the two. New solutions, such as small cells and distributed antenna systems (DAS), have been added to each mobile generation to improve the performance and quality of experience (QoE) for indoor users. However, the goalposts are moved with each new generation of use cases, and even higher levels of performance and QoE are required.
The improvement in the indoor experience needs in the 5G era must be considerable. The 5G standards enable the networks to support huge numbers of users or connected devices in small spaces (up to 1 million per square kilometre), while consuming data at multi-gigabit speeds and with very low latency and high reliability.
These capabilities, and others, greatly expand the potential business case for 5G operators. They will be able to deploy a fully mission-critical industrial network for the first time, and can use the same technology to support high-definition video content for thousands of consumers in a stadium or concert hall. For example, the 5G network in a major transport hub (such as Shanghai Railway Station) needs to support 330 000 people each day, consuming a total of 17 000GB of data. Machine vision applications are increasingly being introduced into large factories and these can involve 50–60 cameras that require as much as 2Gbit/s capacity per 1000km2.
Traditional solutions are reaching their limits when it comes to capacity and uplink performance
The 5G use cases outlined in the previous section will greatly enhance the revenue opportunity for operators, as well as the experience for users and enterprises. However, the solutions that delivered strong indoor QoE for 4G-era use cases will not always be able to achieve the same results in these advanced usage scenarios.
Cell splitting is the most common approach to improving capacity and throughput in in-building networks. This involves splitting a single indoor cell into several smaller ones to improve spectral reuse and targeted capacity. This can deliver a marked capacity gain (a gain of about a third would be typical in a three-cell split), which would support improved QoE in many use cases. However, such gains will be inadequate in 5G scenarios where multi-gigabit throughput and very high capacity are required.
The main limitation of splitting is the interference between neighbouring cells. This leads to a sharp drop in throughput for users or connected objects at the edge of a cell. Data-channel interference drives down the signal-to-noise ratio (SINR), which results in lower cell throughput; a 7dB drop in SINR, for instance, can reduce throughput by almost 50%. This, in turn, cancels out the capacity benefits that can be obtained from advanced radio features such as high orders of modulation (256QAM).
The consequent deterioration of the QoE can be problematic for people in a stadium or airport, but is disastrous in an industrial environment where the rate and reliability of the connection must be absolutely guaranteed, on the uplink as well as the downlink. For instance, applications in a manufacturing environment often require a deterministic network rate, otherwise production can be disrupted. In Guangdong Electronics Assembly factory, for example, 8 HD cameras are irregularly distributed across a 1000km2 production plant to perform AI-enabled quality inspection. Each camera requires 75Mbit/s in consistent and reliable uplink throughput wherever it is positioned in the plant.
An operator’s KPIs may be adversely affected if they cannot address critical enterprise applications of this nature, or if their consumer customers have a sub-standard experience.
Distributed massive MIMO promises a significant improvement in indoor capacity, throughput and QoE
Distributed massive MIMO is an emerging solution to optimise 5G capacity, throughput and QoE indoors. Massive MIMO is usually associated with outdoor base stations, whose capacity is boosted by using arrays of 16 or more physical antennas combined with spatial multiplexing and beamforming. The same concepts can be applied in a different way to optimise indoor performance. In this case, the multiple antennas are logical rather than physical, and are geographically distributed over the cell so that a user will always be close to at least one antenna. This promises several advantages.
- 4T4R is the upper limit for conventional indoor base stations (using massive MIMO technology) because of size and weight constraints. Distributed massive MIMO combines multiple distributed antennas into a single logical cell and so can match the capacity of a macro active antenna unit (up to 64T64R).
- Creating a single cell with many antennas distributed throughout it will also address the interference problems found with cell splitting, and spatial multiplexing and beamforming will further improve QoE.
- User experience is enhanced by joint beamforming in locations where the ranges of two or more antennas overlap. This enhances capacity and enables the cell to take maximum advantage of high-order modulation. Capacity is increased at the cell edge, where only one antenna is in reach of the user, because of the lower interference and through multi-user MIMO techniques. Multi-user MIMO pairs devices on the uplink and downlink to increase cell capacity.
- In trials that compared distributed massive MIMO with four-way cell splitting, the former delivered data rates that were up to 300% higher on the downlink and 200% higher on the uplink. Peak cell capacity was 3–4 times higher on uplink and downlink for distributed massive MIMO.
The distributed massive MIMO approach will therefore have a great impact on the user experience and operator business case, even in the most demanding indoor 5G environments. As noted above, these typically need to support either very large numbers of users with high data requirements (for example, transport hubs, stadiums or shopping malls) or must have extremely reliable levels of uplink and downlink performance (as required in industrial environments, for example). Indeed, these example locations are exactly where the first commercial deployments of distributed massive MIMO have taken place. For instance, China Unicom achieved a peak cell throughput of 4.7Gbit/s in the exhibition hall of the National Centre for the Performing Arts in Beijing. A peak of just 1.3Gbit/s would be expected if 4T4R cell splitting were used instead. In this deployment, individual users received speeds of 1.2Gbit/s in areas of antenna overlap.
Such performance gains have a direct impact on an operator’s competitiveness and 5G return on investment. For example, LG U+ has deployed distributed massive MIMO in locations in Seoul, South Korea such as hospitals and metro stations and has reported downlink speeds of up to 1.1Gbit/s per user. This is a 25% improvement on scenarios without this technology in use. This has enabled the operator to deliver highly competitive services even though it has a spectrum disadvantage in the 3.4–3.7GHz 5G band (it has 20MHz less spectrum in this band than its two competitors). It is delivering a per-user data rate of 1Gbit/s everywhere using 80MHz of spectrum, which is 40% higher than its competitors are able to achieve using 100MHz of bandwidth.
Many operators are in a similar position to LG U+. In very competitive markets, they need to expand their business cases to advanced consumer and enterprise services in order to increase their revenue and enhance customer satisfaction. However, they may not always secure the ideal amount or type of spectrum, or their customers may have particularly challenging indoor environments in which to support a strong user experience cost-effectively and consistently. Distributed massive MIMO will be a valuable addition to the range of 5G solutions, whatever the individual challenges faced by each operator are.
Looking ahead, there will be further evolution of the concepts that underpin the first generation of distributed massive MIMO systems. An important trend in cellular architecture is to plan the network around the user, rather than the cell, in an increasingly dynamic way that aligns capacity to the needs of a user or application at a particular point in time. This will be increasingly important in delivering high-performance indoor coverage, and will eventually lead to a fully user-centric, no-cell (UCNC) network, with a dramatic impact on each user’s quality of experience.
This article was commissioned by Huawei. Analysys Mason does not endorse any of the vendor’s products or services.
Article (PDF)
DownloadAuthor
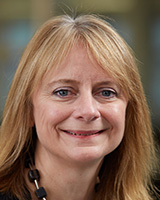