Hollow-core fibre for low latency and increased bandwidth: the next game-changer in optical cables
27 September 2022 | Strategy
Article | PDF (3 pages) | Fibre Infrastructure Strategies
Listen to or download the associated podcast
The amount of data carried through telecoms networks is increasing at a rate of between 20% and 30% every year. This is partly driven by the move to next-generation access (NGA) and higher-speed mobile access networks. Hyperscalers and cloud players are also creating new demands for high-capacity core networks between data centres.
In addition to the apparent need for higher capacity over time, specific applications such as high-frequency trading, gaming or augmented/virtual reality (including the metaverse) have very low latency requirements.
Network operators have been addressing the trend of increasing demand by increasing the optical transmission capacity of their fibre networks. Each wavelength is carrying more data, and some networks are using dense wavelength division (DWDM) (that is, more wavelengths per fibre). However, some operators and hyperscalers are reaching the limits of silica-based fibre technology and to meet higher demand will eventually have to light or deploy additional fibres to cope with the demand. An alternative is to shift to radically different optical transmission medium technology called hollow-core fibre.
Silica-based fibres impose latency limits and restrict the use of spectrum to C and L bands for long-haul applications
Optical transmission has revolutionised telecoms by enabling the transmission of terabit per second bandwidth over a single fibre pair over long distances (for example, thousands of kilometres in submarine applications). However, the fibre medium has traditionally been based on fused silica (glass), which has two limitations.
- Latency. Silica has a refractive index of (roughly) 1.5 and optical electromagnetic waves can only be transmitted in a silica medium at two thirds of the speed of light in a vacuum. This is in marked contrast with systems that use air as a transmission medium (for example, microwave links) where the signal can be transmitted at close to the speed of light in a vacuum (‘c’).
- The attenuation profile limits specific spectrum transmission windows. Traditional silica-based fibres can only use a small spectrum window to transmit signals over long-haul applications, due to the need for low attenuation. If the attenuation was lower over a wider spectrum window, additional spectrum could be used in the fibre, and significantly higher bandwidths could potentially be carried on each fibre (provided that suitable electro-optic devices are developed to match these as-yet untapped spectrum bands).1
The latency of optical fibre is adequate for most current applications, but some applications are highly sensitive to latency. For example, in high-frequency trading applications, if the signal must travel between Frankfurt and London (700km), and all else is equal, a trader using a fibre-optic system between these two locations will receive the latest stock quotes around 1 millisecond after a trader who uses a microwave transmission system giving the latter an advantage (and indeed microwave systems joining London to Frankfurt in a straight line have been built for this very reason). Systems that cross major oceans or continents face even larger impacts.
The transmission of optical signals over long distances is designed to use the C band and L bands (Figure 1) – the so-called ‘third transmission window’. This is because signal attenuation is wavelength-dependent and the third transmission window wavelength range exhibits the lowest attenuation.2 Up to the L band, the floor in attenuation is dictated by Rayleigh scattering (as light travels in the core, it interacts with the silica molecules and these collisions between the light wave and the silica molecules result in attenuation or loss of signal), which is an inherent property of silica-based fibres. For wavelength in the U Band and above, the attenuation becomes dominated by absorption.
Figure 1: Silica-based fibre attenuation profile as a function of wavelength
Hollow-core fibre can transmit signals at a higher speed than silica-based fibre and could release additional bandwidth
To avoid these limitations, significant focus has been recently put on researching hollow-core fibre, which transmits the signal in a very small tube filled with air (instead of silica/glass). Although the significant improvement in latency of hollow-core fibre over silica-based is well-established (that is, transmission at the full speed of light), hollow-core fibres have traditionally suffered from higher attenuation than silica-based fibre.
One of the reasons for the higher attenuation is due to manufacturing process challenges to produce these hollow-core fibres, which are still at a nascent stage of their lifecycle. However, if the manufacturing processes keep on improving, hollow-core fibre could be produced with significantly lower attenuation than silica-based fibre, overcoming the fundamental Rayleigh limit in the silica-based medium. In turn, lower attenuation would enable:
- transmission over longer distances, reducing the requirement for optical amplifiers and repeaters or allowing the use of lower-powered transmitters, with resulting power savings
- additional spectrum bands to be used for transmission in long-haul applications, which would allow significantly more capacity per fibre.
Commercial deployments of hollow-core fibre are starting for low-latency applications but are still at a nascent stage
euNetworks, a Western European bandwidth infrastructure company, announced in April 2021 that it had deployed the first hollow-core fibres from provider Lumenisity.3 The link is between Interxion, a data-centre provider and the London Stock Exchange. This was the first commercial deployment of this technology in the world and was part of an agreement covering additional deployments over the coming years between trading venues and other locations.
Comcast announced in April 2022 that it had deployed a 40km hollow-core fibre link in its home market of Philadelphia.4 The fibre provider was also Lumenisity. Comcast tested bidirectional transmission on the same fibre strand at speeds of 10Gbit/s to 400Gbit/s. Comcast says 40km is significantly longer than previously demonstrated hollow-core fibre deployments. Comcast’s core business is residential rather than commercial services, so the most likely benefit of ultra-low latency is supporting gaming or other immersive consumer applications (such as VR/AR) that the metaverse might bring.
Hollow-core fibre roll-out will address low-latency needs at first and may occur at scale in the late 2020s if attenuation can be reduced
Use of hollow-core fibre will require operators to install new fibre cables that can reside in the same ducts as existing silica-based fibre. We expect more operators in developed markets to test hollow-core fibre in 2023 and limited commercial deployment in 2024 and beyond to complement existing fibre/microwave links in handling low-latency requirements. As the technology evolves and, if we are correct, that attenuation in hollow-core fibre becomes lower than that of silica-based fibre, additional currently unused spectrum will become available, allowing greater capacity per hollow-core fibre. Therefore, we do expect larger-scale deployment of hollow-core fibre in core networks in the late 2020s/early 2030s.
1 Some of these spectrum bands are used by coarse wavelength division multiplexing (CWDM) applications but cannot transmit over large distances.
2 Erbium-doped fibre amplifiers (EDFA) have also been developed to specifically operate in the C and L bands to extend transmission reach.
Article (PDF)
DownloadAuthor
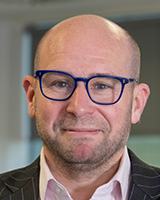
Franck Chevalier
Co-Head of TechnologyRelated items
Article
Future fixed traffic demands depend less on technological leaps and more on user engagement
Forecast report
Fixed network data traffic: worldwide trends and forecasts 2023–2029
Article
Recent M&A activity shows that delayering is now affecting FTTP delivery in Asia and North America