Why LEO’s Target Fixed and Mobility Markets Simultaneously
Authors: Christopher Baugh | Carlos Placido (independent adviser)
Satcom constellations beaming tens of Tbps are impacting the space sector and the broader telecom industry. Yet, when running demand-supply simulations on fixed-broadband and mobility datasets using NSR’s Non-GEO Constellations Analysis Toolkit 2.0 (NCAT2), it becomes clear that, while the amount of IP bandwidth produced is enormous, not all is usable or sufficient.
Two key aspects need to be considered simultaneously when analyzing bandwidth supply/demand dynamics and even future pricing strategies of LEO mega-constellations:
- Hundreds to thousands of satellites orbiting at low altitudes produce high levels of frequency-reuse 'spotbeam' HTS capacity.
- However, LEO’s limited visibility of the Earth’s surface reduces addressability for all such bandwidth supply.
The combination of these two aspects leads to highly localized supply-demand dynamics and even buy/sell opportunities that –both– satellite operators and educated users or distributors could leverage with various degrees of bargaining power. This also means that LEO operators absolutely need to target fixed-data and mobility markets (aero/maritime/rail) simultaneously to maximize addressability and fleet-wide fill rates. Maximizing addressability is key towards the ultimate goal of minimizing the capital cost per usable Mbps, which is core to constellations’ business case and long-term sustainability.
NSR’s NCAT2 provides tools and datasets to assess supply and demand factually for fixed-data and mobility applications -independently or combined- per degree of latitude and longitude. A dashboard tool included in NCAT2 facilitates the assessment at global, regional or territory levels (countries selectable individually or in groups).
Sample Simulation: Starlink
The sample simulation below corresponds to SpaceX Starlink, considering two constellation shells fully deployed. For simplicity, this assessment assumes that SpaceX has “open skies” access to all visible territories worldwide for fixed-data and mobility markets (details below),but note that NCAT2 allows the analysis to be conducted for a selectable list of countries. As an example, China and Russia markets may be excluded if simulation needs to assume that SpaceX will not have access to such territories. Any combination of included/excluded countries can be selected in the tool.
General assumptions for the sample-case analysis:
- Supply: Starlink sub-constellations (shells) 1 and 2 fully deployed.
- Demand: Rural fixed-broadband plus aero mobility (details below)
Bandwidth Supply Model
The bandwidth supply model assumes full deployment of Starlink shell 1 (53°
Inclination shell; now completed by SpaceX) and shell 2 (70°inclination shell; in the process of being launched). User beam and satellite IP throughput are calculated in the toolkit (Tool #5 “Throughput Link Budgeting”) based on technical characteristics extracted from publicly available FCC filings and other assumptions (antenna size, look angles, margins, etc.). NCAT2 Tool 4 (“Satellites in of Sight”) was leveraged to calculate the number of visible satellites based on latitude, as shown below.
Starlink shells 1 and 2 together comprise over 2,300 satellites beaming 21 Tbps (terabits per second) of user-beam Ku-band capacity globally. By virtue of orbital mechanics, such capacity is not distributed evenly as the number of visible satellites drives local supply density. The map shows the density of capacity supply that is proportionally overhead for the combined two-shell system.
Fixed-Broadband Bandwidth Demand Model
This simulation assumes that target regions for fixed broadband services are those with a population density between 5 and 300 inhabitants per square kilometer (13 to 777 inhabitants per sq.mile). Areas with less than 400 inhabitants per sq.km are considered rural areas, and generally targeted by satellites (Non-GEO and GEO). A total of 3.48 billion people live in such regions (the “other 3 billion”), representing the global addressable population.
The model assumes a market capture for Starlink of 1% of such population (also editable), so roughly 35 million people are assumed connected to the Internet via the two Starlink sub-constellations. The simulation considers a 50/5 Mbps (downstream/upstream) service plan with a 40-to-1-contention ratio (overbooking). Note that this analysis process can agnostically apply to scenarios combining (direct) residential broadband users and local mobile users reached indirectly via MNO wireless backhaul.
Mobility Demand Model
NSR used NCAT2 Tool 12 (“Mobility Heatmap”) to run a simulation of in-flight entertainment and connectivity (IFEC) demand for a few major airlines, namely American Airlines, Air France, British Airways, Turkish Airlines, Ryanair and three major Chinese airlines. Data for airline routes was extracted from OpenFlights.org. Such data is not updated but nevertheless useful for this example. NSR produced “dummy” flight schedules for the airlines based on routes and average flight speed. Simulation was run across a 24 interval, so the number of aircraft present per degree of latitude and longitude shown below is the total day count.
For the combined demand analysis, the model assumes a service plan of 100/20 Mbps per aircraft without contention (committed information rate; all editable inputs in the tool).
Demand Assessment of Combined Fixed-Broadband and IFEC
Calculation of combined broadband and aero (IFEC) capacity demand is based on the respective service plans described above, and the geographic distribution. For the combined bandwidth demand map, the number of aircraft per degree of lat/lon is the one from the heatmap tool divided by 48. This is because the aero heatmap simulation was for 24 hours so, assuming 12 hours of "peak flight time"; this would give a more realistic picture of the number of aircraft per lat/lon degree, per 15-minute time interval.
The global aggregate demand equals 62 Tbps, of which 48 Tbps correspond to fixed broadband, based on the configured inputs. Demand exceeds supply globally, but it is concentrated in certain hot spots, as analyzed next.
NCAT2 Model Output: Supply & Demand Heatmap Analysis
The NCAT2 Dashboard tool compares supply and demand of bandwidth per degree of latitude and latitude to drive a "Supply & Demand Heatmap” analysis: Red dots in the map denote 1 deg. lat/lon spots where demand is higher than supply; and green areas are those where demand is lower than supply. Pure yellow areas illustrate a rather balanced supply and demand.
Supply is measured as "proportional overhead capacity" in the tool. Since there are multiple satellites visible, red spots could turn yellow (or green) if capacity is redirected or "stolen" from neighboring areas with lower demand. Operators’ orchestration systems and bandwidth policing will certainly drive network optimization but note that user beam steerability does not necessarily eliminate all red spots because of the small field of view of the LEO satellites (hundreds of km radius) and operator’s need to comply with the minimum exclusion angles required by the ITU to avoid interfering with GEO Ku band satellites, particularly around populated Equatorial regions (assessed separately in another NCAT2 tool).
The NCAT2 dashboard tools provides summarized metrics for the analysis and detailed supply and demand data tables (per degree of lat/lon) in case users need to export data for use outside the NSR toolkit.
Bottom Line
Sir Arthur C. Clarke, who conceptualized the geostationary (GEO) communications satellite, once made a wise comment that –paradoxically– today applies to the LEO addressability dilemma: “How inappropriate to call this planet Earth when clearly it is Ocean”. Roughly two thirds of Earth is ocean. In the sample case analyzed above, the addressable fixed-broadband population is distributed across a surface area that represents only 12.5% of the world surface (land and water), showcasing the absolute must for LEO operators to expand addressability towards mobility routes (aero/maritime/rail), so as to drive a more efficient business case.
Analysis also showcases why mega-constellations plan to deploy additional sub-constellations to expand bandwidth supply within 35 degrees, north and south to the Equator, where 75% of the addressable rural population resides.
NSR’s Non-GEO Constellations Analysis Toolkit 2.0 (NCAT2) is an assembly of flexible, configurable, and easy-to-use quantitative models that x-ray and benchmark LEO/MEO high throughput satcom constellations at architectural and business layers. The toolset provides a data-driven, unbiased vehicle for deep-diving into the intertwined technical and business aspects driving bandwidth supply, addressability and feasibility of leading mega-constellations, and their competitive standing versus terrestrial networks.
Benchmark SpaceX Starlink, SES O3B, OneWeb, Telesat Lightspeed, Amazon Kuiper, and (any) other LEO/MEO satellite system using the NSR toolbox. NCAT2 includes a multiplicity of analytical tools distributed across 15 Excel files (plus user guide and mobility datasets). Each tool has its own set of input variables, filters and calculation engines that drive output results, charts, maps, exportable data tables and visualizations.
Author
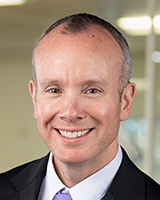